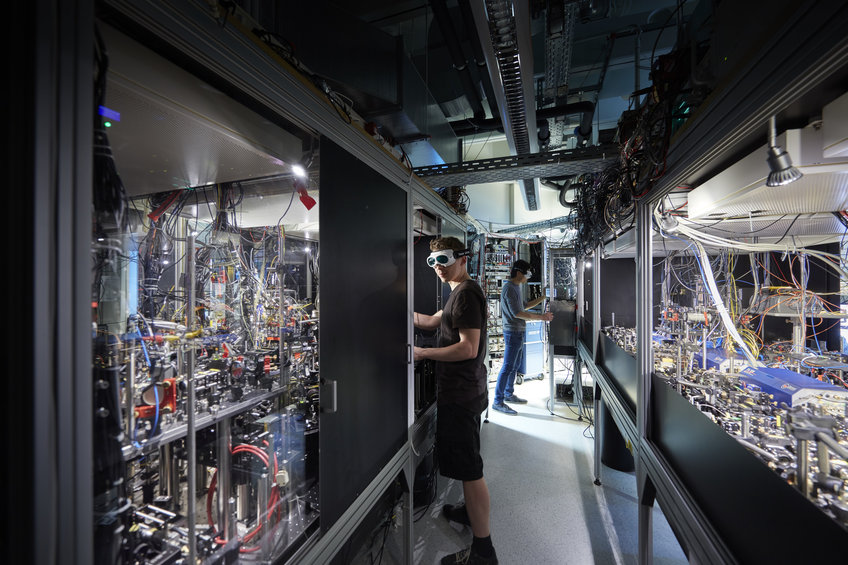
The Rubidium Quantum Gas Microscope
In our 87Rb quantum gas microscope we are able to detect and manipulate Rubidium atoms in optical lattices with single-site resolution and single-atom sensitivity. Our work focuses on exploring long range interacting Rydberg gases and probing non-equilibrium phenomena such as many-body localization in this unique setting.
A subradiant atomic mirror
Versatile interfaces with strong and tunable light–matter interactions are cornerstones for quantum science. Recent proposals have suggested a fundamentally new approach to realize such systems by using the rich interplay of photon-mediated dipole–dipole interactions in structured subwavelength arrays of quantum emitters: First, the close spacing between the atoms enable efficient exchange of photons between the atoms, such that the atoms can couple to the optical field in a cooperatively enhanced way. Second, the periodic subwavelength ordering of the atoms leads to directional emission of the light field – similar to classical optical gratings, where higher-order diffraction is suppressed. In this work, we report the first demonstration of the key aspects of this approach, the cooperative enhancement of the light–matter coupling strength and the directional mirror refection of incident light. Furthermore, we observe the cooperative subradiant optical response of the entire system, where the spectral width of the collective excitation reduces to a value below the quantum-limited natural linewidth of individual atoms in free space. By controlling the atom density or the spatial geometry, we elucidate the effect of the interplay of spatial order and dipolar interactions on the cooperative response of the system. Our work demonstrates efficient optical meta-material engineering based on structured ensembles of atoms and paves the way towards controlling many-body physics with light and novel light–matter interfaces at the single-quantum level.
Floquet prethermalization in a Bose-Hubbard system
The periodic driving of both classical and quantum systems can reveal new phenomena without an analog in non-driven systems. Nonetheless, periodically driven systems do not fulfill energy conservation, which means that many-body systems will absorb energy from the drive and, eventually, heat up to an arbitrarily high temperature, what is known as "heat death". A way around this is to postpone the inevitable by driving the system at sufficiently high frequencies, which has been predicted to exponentially suppress the heating rates. In this experiment, done in collaboration with theorists from Stanford and Princeton University, we use our quantum-gas microscope to observe an such an exponential suppression of the heating in a cloud of ultracold bosons. Our results open the door to the creation of novel non-equilibrium phases of matter, and provide new understanding on the heating processes taking place in driven many-body system.
APS/Alan Stonebraker
Long-range Rydberg interactions
Interactions in cold atomic systems are usually short ranged. Rydberg atoms offer the possibility to realize very strong, effectively long range, interactions. We study the resulting long range interacting quantum spin models experimentally using our microscope. One unique feature of our approach is the direct imaging of the Rydberg excitations in the interacting many-body system. Using this technique we were able to study spontaneously ordered quantum states of Rydberg excitations. Among our most recent works, we have studied the Ising-like interactions emerging from Rydberg dressing and observed the photoassociation of giant molecules, so-called Rydberg macrodimers.
Out-of-equilibrium dynamics in clean and disordered systems
The thermalization of an isolated quantum system can fail in the presence of quenched disorder, even with interactions. This phenomenon, known as many-body localization (MBL), has been recently the focus of much theoretical work, though many open questions still remain regarding its existence in higher dimensions or its robustness to a finite bath coupling. Ultracold atoms in optical lattices have emerged as an extremely suitable platform for the study of MBL, and promise to shed light into some of its properties.
In our experiment, we use a quantum-gas microscope with projected disorder to study the dynamics of a quenched state of bosons in two dimensions, where we observe a remaining memory of the initially prepared state by measuring the evolution of its imbalance. By introducing a second bosonic species unaffected by the disorder potential, a thermal component has been added to the system, and we have measured its effect on the disordered component, which in the presence of a big enough thermal component ultimately loses its imbalance.
Group members
- +49 89 3 29 05 - 677 (Office) // - 219 (Lab)
- +49 89 3 29 05 -677 (Office) // -219 (Lab)
- +49 89 3 29 05 - 238 (MPQ)
- +49 89 3 29 05 - 218 (Office) // -219 (Lab)
- +49 89 3 29 05 - 229 (Office) //-219 (Lab)
- +49 89 2180 6151 (LMU Office) // -6136 (LMU Lab)
Recent publications