Ultracold atoms in a honeycomb optical lattice
In this experiment, we use ultracold atoms in a graphene-like honeycomb lattice to realize a clean and highly tunable system in which to probe topological effects that are difficult to study in solid state systems.
Experimental setup
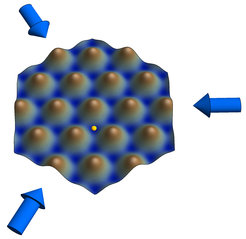
Our setup includes Potassium an Rubidium, such that we can work with bosons or fermions and have readily accessible Feshbach resonances. In our setup, we first cool the atoms in a 2D-MOT. The atoms are then further cooled in a 3D MOT before being magnetically transported to the science cell. Here, we reach quantum degeneracy through forced evaporation first in an optically plugged quadrupole trap, followed by further evaporation in a crossed dipole trap. The atoms are then loaded into the honeycomb lattice, which is created by superimposing three blue-detuned running waves at 120 degree angles to each other (arrows). The atoms are loaded into the minima (blue) of this potential landscape and can quantum-mechanically tunnel between them. One possible position is illustrated by the small sphere.The resulting band structure mimics that of graphene, with Dirac cones located at the corners of the Brillouin zone.
An Aharonov-Bohm interferometer for determining Bloch band topology
The energy band in a solid is characterized not only by its dispersion relation, but also by its geometric and topological properties, which are fundamental for a wide range of many-body phenomena in condensed matter, such as the integer quantum Hall effect. We have recently implemented a new type of atom interferometry in order to study the Berry curvature of the lowest band of the graphene like band structure.
In an Aharonov-Bohm interferometer, a magnetic field confined to the interior of a solenoid affects the behavior of electrons outside it, shifting the phase of their wave-like interference although they never directly encounter the magnetic field. This classic experiment is a striking demonstration of the non-local character of quantum mechanics. In the Berry-flux interferometer implemented in our work, a conical intersection of energy levels—known as a Dirac cone—plays the role of the solenoid in the interferometer. Even though the graphene bands are characterized by a vanishing Chern number, the individual Dirac points located at the corners of the Brillouin zone are still topologically interesting. We have studied the geometric properties of this band structure using interferometry with a BEC of 87Rb, which allows for a momentum-resolved probe.
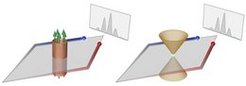
Bloch state tomography using Wilson lines
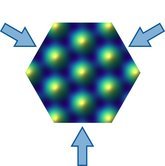
The geometric and topological structure of energy bands play an important role in modern condensed matter physics but are difficult to experimentally access. Using ultracold bosons in a honeycomb lattice, we demonstrate a straight-forward method that can be used to both reconstruct the Bloch states at every quasimomentum and determine the topological invariants of the bands. Our method is based on using strong-force dynamics to realize a system that can be described by Wilson lines.
Interaction dependent heating and atom loss in a periodically driven optical lattice.
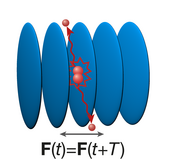
The periodic driving of optical lattices has become a versatile tool to create novel band structures not realizable in static lattice systems. However, especially interacting bosonic systems often suffer from strong heating which impairs the observation of the physical properties of the created system. In order to find strategies to avoid this heating in future experiments, one first has to identify the underlying heating mechanisms. To this end we studied interaction dependent loss rates of bosonic atoms in a periodically driven one-dimensional optical lattice. We identified a variety of intra- and interband resonant scattering processes that depend on both the scattering length and the driving strength. We find that heating rates are dramatically reduced when shaking with a frequency larger than the trap depth.
In this regime scattered particles can leave the trap before dissipating their energy into the system. As the identified scattering processes rely on at least one weakly confined transverse direction, one powerful strategy to reduce heating even further might be the implementation of shaking in a three-dimensional lattice structure.